Design for Energy—Framework for Design Excellence
Good design reduces energy use and eliminates dependence on fossil fuels while improving building performance, function, comfort, and enjoyment.
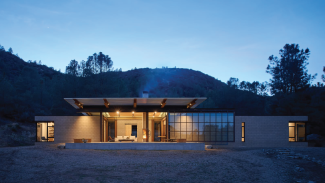
- How can passive design strategies contribute to the project’s performance and form?
- How can the project exceed building code efficiency standards to approach net zero energy and net zero carbon?
- Can the project be powered by clean, renewable energy sources?
- How can the project provide for continuous performance improvements over its lifetime?
Focus topics
- Passive design strategies
- Energy benchmarking & goal setting
- Eliminating fossil fuels
- Energy modeling
- Commissioning
- Net zero energy (NZE) building
- Net zero carbon (NZC) building
- Building grid integration
Design for Energy toolkit
If you can do only one (or a few) thing(s):
- ZERO CARBON: Reduce building energy use and carbon impact by benchmarking, setting targets, and performing iterative energy modeling throughout the design process.
- RESILIENT: Design all-electric buildings with grid interoperability to take advantage of distributed energy sources.
- EQUITABLE: Design to achieve passive survivability without the use of grid energy. This supports low-income residents by reducing operating costs and providing safety in the event of grid interruption or termination.
- HEALTHY: Transform energy use by promoting renewable energy and embracing building electrification to improve indoor air quality for occupants.
The resources in this toolkit focus on what the architect can do. For example, although passive measures are usually the sole responsibility of the architect, impacts to other systems, such as lighting and HVAC design, are inevitable and fall within an architect’s scope.
Passive design strategies
High-performance design engages the entire design team and the client and should be conducted as early as possible. Building performance can be greatly impacted with simple, climate-specific, passive strategies at the conceptual design phase, such as building orientation, window-to-wall ratio, and exterior shading.
Actions:
- Indigenous and native typologies offer great clues for climate-responsive design. Explore the vernacular and indigenous architecture as a guide to determining the passive strategies that are most applicable to a given region.
- Establish an optimum building orientation for the project. Locate the building on the site to take advantage of the way the sun moves across the sky; typically, this should mean that the longest façade of the building should have exposure to north and south, and shortest façade to east and west.
- Focus on window-to-wall ratio (WWR), orientation of glazing, and sun shading as major passive strategies. WWR should be limited in all climates, but, in general, the location of glazing will shift depending on the latitude. In colder climates in the Northern Hemisphere, primary glazing should be on the south to collect beneficial solar radiation. In warmer climates in the Northern Hemisphere, primary glazing should be on the north to avoid harsh summer sun. If the project is in the Southern Hemisphere, the guidance for north and south glazing should be switched. For the most part, windows should be shaded on the south, east, and west (in the Northern Hemisphere) and minimized on the east and west in all climates. Consider the program of the rooms along the perimeter and how they may benefit from daylight harvesting lighting controls, which benefit the occupants and offer potential interior lighting energy savings.
- Be mindful of the shape of the building; a compact form is more energy efficient than a sprawling one for small- and medium-scale projects. A building with an extended outer surface will lose more heat (in cold climates) or gain more heat (in warm ones).
- Envelope air tightness is just as important as insulation but often receives less attention. Designate one layer of the assembly as the air barrier and confirm that this layer is continuous in all directions on six sides, with all seams taped and all penetrations filled. Use envelope commissioning or a blower door test to verify the building's air tightness, both for mockups and for the whole building.
- The amount of insulation prescribed in the building codes is the minimum. However, additional insulation can reduce the peak load/mechanical size or improve resiliency for many buildings. Analyze building assemblies to determine the amount of insulation that will provide thermal comfort and efficient energy performance without a diminishing return based on the increased capital cost.
- Thermal mass can be used to absorb energy during the day and release it in cooler night hours in places with diurnal (day/night) temperature shifts.
- Provide operable windows for all occupants so that the building can benefit from outdoor air when the weather is agreeable and exterior air quality is at safe thresholds. An interlock between window opening and mechanical system prevents loss of treated air. In terms of resilience, operable windows will aid in ventilation and air quality if the utility grid is down and building mechanical systems are offline.
Energy benchmarking & goal setting
This is a critical first step for every project. See ASHRAE 90.1 for early modeling guidance.
Actions:
- At the beginning of the design process, establish benchmarks and set an energy use intensity (EUI) goal and any other key performance indicators, such as carbon emissions. Benchmarks are easily shared with the design team and owner and are a basis for a deeper conversation about how the building is intended to work. Everyone on the team should understand a project’s benchmark and its energy goals.
- Establish a benchmark and set a lighting power density (LPD) goal at the beginning of the design process. LPD is measured in watts per square foot (W/sf) of installed lighting. This is a great indicator of a project’s energy performance. Projects with a lower LPD will use less energy. Holistic lighting design should consider all sources of illumination—daylight, overhead lighting, and task lighting—to find a balance that keeps the base lighting load as low as possible.
- For interior projects (including projects with lighting design; HVAC system modifications or substantial building envelope modifications), set a goal for LPD of 25%–50% better than code. Check the LPD often throughout the design process.
- When evaluating various buildings for a potential tenant, consider the daylight penetration to the floor plate, and design to include as much regularly occupied floor area within the daylit zone. Interior material finishes can enhance daylight quality or exacerbate interior glare and discomfort based on the contrast or reflectivity they provide to the occupant’s field of view.
- When evaluating various buildings for a potential tenant, consider the daylight penetration to the floor plate, and design to include as much regularly occupied floor area within the daylit zone. Interior material finishes can enhance daylight quality or exacerbate interior glare and discomfort based on the contrast or reflectivity they provide to the occupant’s field of view.
- The optimal window-to-wall ratio (WWR) will set the stage for both good daylighting and energy performance. Windows are a major indicator of total building energy use. A WWR above 40% will provide no additional benefit for daylighting but will cause significantly higher conditioning loads.
- Architects can also consider top lighting strategies to provide daylight in buildings with a wide floor plate.
- Analyze the project plug load (in W/sf). Very efficient buildings tend to have a greater percentage of the energy coming from plug loads. Like LPD, it is important to set a goal for plug loads and check them throughout the design process. Determine the typical plug load (in W/sf) for buildings with a similar program and aim for a 25% to 50% reduction. Scheduling nonessential plug loads to turn off when not in use can be a primary strategy for reaching 50% reduction.
Eliminating fossil fuels
Electrification functions as a decarbonization strategy when electricity is generated with clean resources such as wind and solar power. More areas of the country are moving toward low-carbon energy generation for the grid. To reach carbon targets, buildings must shift to electric or district heating and cooling systems powered by carbon-free renewable energy sources. Electrification in buildings means using electricity for all the core functions such as heating, cooling, and cooking.
Beneficial electrification reduces energy costs for consumers, reduces harmful emissions across the entire supply chain, and is coordinated with peak demand reduction.
Actions:
- Check for regulatory requirements limiting fossil fuels. Many communities across the country are restricting on-site fossil fuel use.
- Pair efficiency with electrification to ensure low operational costs.
- Utilize ground-source heat pumps, air-source heat pumps, high-efficiency energy recovery units, and other equipment with significant energy performance improvements. These innovations make electrification viable for most projects.
- Specify induction cooktops and stoves. This has proven health benefits for indoor air quality.
- Assist clients by guiding them to grants (which may be available from utilities or government sources) to offset the transition cost from gas to electricity.
Energy modeling
The AIA 2030 Commitment clearly demonstrates the relationship between energy modeling, high performance, and effective operational carbon emission reduction. When an energy model is performed, higher performance is a typical outcome. While energy modeling can be a very specialized skill set, the architect should always oversee this process.
Project teams should consider engaging an energy modeler during the predesign and concept stages. A high-level modeling approach will work well when performance iterations need to be completed alongside design conversations. Let the client know that performance modeling results are fuzzy at best and are intended to set the project direction and help the architectural team in making design decisions before a concept has been developed. The scope can vary depending on project size, complexity, and budget.
Energy modeling can be done to varying degrees of detail and scale. A simple box model performed early in the project will highlight the sensitivity of passive strategies, such as building massing and orientation, fenestration (including consideration of orientation and various window-to-wall ratios), shading options, and thermal performance of the building envelope. As the design progresses, a more detailed model can aid in identifying load reduction strategies, such as reducing interior lighting and equipment loads, ventilation strategies, and HVAC system options. Depending on the complexity of the project and the scope of the model, this detailed energy model can take anywhere from half a week to two weeks.
Energy modeling to demonstrate compliance with building energy codes or other accreditation programs (e.g., LEED, Living Building Challenge) will require the expertise of an energy modeling professional with approved software as determined by ASHRAE Standard 140. Demonstrating compliance with an energy model with a performance-based path provides an architect with greater flexibility in design, as performance tradeoffs are considered dynamically between the building envelope and MEP design.
Actions:
- Build iterative energy modeling into your project’s design budget. Whether the work is done in-house, in cooperation with a utility program, or with a consultant, there are many ways to integrate analytics that inform the design into your project.
- Consider the energy model as a cost-control measure, not as an add-on for sustainability. Energy and carbon are relatively easy to quantify and predict, and the predicted annual results can be used to manage capital costs (system plant size), operational costs (utility bills), and other non-energy benefits (NEBs) such as thermal comfort.
- If the scope of the project is too small for an energy model, manage the window-to-wall ratio and lighting power density. These simple calculations are excellent proxies for energy modeling.
- Look closely at occupancy schedules, occupant densities, operational hours, and physical form. Much of a building’s energy and carbon use is tied to the building’s function and climate zone. Understand how these variables affect what measures are employed.
Commissioning
Building commissioning (Cx) is incredibly important in ensuring that the owner receives the building they paid for. Cx can be expensive but tends to pay back quickly and provides valuable quality assurance/quality control. The scope can vary greatly and should be carefully coordinated with the systems being proposed in design. Ensure that any mandatory commissioning requirements from the
local jurisdiction are met. To adhere to the 2012 International Energy Conservation Code (IECC), mechanical, service water heating, and lighting control systems must be commissioned.
Building envelope commissioning (BECx) evaluates the performance of the building envelope to determine if the project requirements have been met. The building envelope is critical to a high-performing building and ensuring the installation meets the project requirements.
Actions:
- Encourage the owner to engage a commissioning agent (CxA) early in the design process to establish test requirements for the project before the testing is performed. The CxA is an independent third party.
- Encourage the use of metering and submetering to measure and track performance across systems and identify areas that are not performing as designed.
- Encourage owners to conduct retro-commissioning to assess system performance after the first three to five years of operation.
Net zero energy building
A net zero energy (NZE) building creates as much energy on-site as it uses through renewable sources (typically photovoltaic [PV] or wind) over the course of a year. A building is not considered to be NZE until after at least a full year of operation when it can be verified that it performs at this extremely high level. Third-party verification or certification is highly recommended.
Zero energy is recognized worldwide as one of the highest aspirations in energy performance in the built environment. The International Living Future Institute’s (ILFI) Zero Energy (ZE) Certification was created for projects to demonstrate zero energy performance, building an advanced cohort of projects with the integrity of third-party performance certification.
Actions:
- Investigate the optimal on-site renewable energy available in your region. Solar energy is a viable option in many areas, while wind is occasionally used at the building scale.
- Design all buildings to be PV-ready in the future. This includes increasing roof carrying load capacities, maintaining large clear areas on the roof or site to mount panels with no obstructions, selecting the correct meters and panels, providing additional capacity for electrical panels, leaving room for inverters, and installing a conduit.
- Metering and data tracking is critical to the building achieving NZE. Consider making data public in the form of a building dashboard to frame goals and progress.
- Incorporate renewable energy in the project. There are many financing options that enable renewable energy to be installed during building construction. Consider a power purchase agreement (PPA) in which a portion of the building or site is leased to a third party for on-site renewable energy. The owner receives the benefits of a low-carbon energy source without the substantial first costs of the renewable systems.
Net zero carbon building
The decarbonization of the built environment is imperative to ameliorate the catastrophic current and future effects of climate change. The industry is at a transition point as we shift from thinking solely about energy efficiency to more holistically thinking about carbon emission reduction.
While net zero carbon (NZC) buildings have many of the qualities of a NZE building, an NZC building goes beyond just energy use and looks at the total carbon emission footprint comprised in both operational and embodied carbon. Operational carbon emissions deal with the building’s sources of energy (such as coal, natural gas, electricity, etc.) and the efficiency of the energy systems in the building. Embodied carbon emissions deal with the impact of building materials life cycles, which include material extraction, production, transportation to the project site, construction application, and end-of-life use.
An NZC building must offset as much operational carbon emissions as it uses over the course of a year. This can include a mixture of on-site renewables and off-site, third-party-verified renewable energy credits (RECs). A challenging goal for any building, NZC may be more accessible for some projects, as project type, shape, size, and access to adequate renewable energy sources determine a building’s ability to meet this goal.
Actions:
- Conduct a whole-building life cycle assessment that includes an assessment of operational and embodied carbon.
- Eliminate the use of fossil fuels by designing all-electric buildings. Provide chargers for electric vehicles (EV) or infrastructure to be EV-ready.
- Prioritize passive design strategies and energy efficiency before adding on-site renewables or considering RECs. A higher priority should be placed on RECs that are local to the project or regionally based. Minimizing the energy used on-site is more impactful than drawing clean energy from a distant source. Plan to achieve NZC with no more than 20% RECs.
Building grid integration
As energy grids utilize more renewables and move away from fossil fuels, they also need to be able to withstand the impacts of extreme weather events and demand surges for power. Building grid integration or grid harmonization is a critical component of decarbonization. It relies on select components within and connected to buildings (inverters, meters, control systems, energy storage systems, renewables) to transition renewable energy to the grid during high-demand periods. Building grid integration requires two-way communication between the building components so that they can manage building load inputs and outputs to the grids and efficiently redistribute power. In certain utility areas, buildings can participate in demand response to help the grid during peak demand. Simple solutions such as grid-integrated water heaters can assist with load shifting during peak demand times. More advanced technologies, such as a microgrid, could provide project sites with the necessary power for short durations (less than four hours) or long durations (greater than 24 hours), allowing a grid to provide stable power to other customers.
Actions:
- Participate in a demand-response program through the local utility. If one isn’t currently available, prepare for demand-response readiness.
- Analyze the building’s annual load shape and peak load. Review the regional grid load profile using the metric of peak load or peak carbon emissions. EPA’s AVoided Emissions and geneRation Tool (AVERT) provides regional grid emissions data.
- Working with the client, develop a comprehensive plan to reduce peak usage by at least 10% when necessary. Identify prescriptive building system components that can provide load flexibility for the grid, such as grid-integrated water heaters that can reduce peak load demands for a few hours or minutes, or renewable systems paired with a battery energy storage system to reduce demand stress and improve grid stability over longer periods.
- Work with your local utility company to determine the carbon impacts and best times for integration to reduce the overall emissions impact. At the building scale, this could involve altering building heating and cooling set points and schedules or timing of electric vehicle charging times.
- The AIA 2030 Commitment offers architects a way to publicly show their dedication, utilizing the Design Data Exchange (DDx) to track progress toward a carbon-neutral future. Read the latest 2030 By the Numbers report to learn more about the industry’s improvements toward 2030 goals.
- The AIA-CLF Embodied Carbon Toolkit for Architects serves to provide architects with an overview and the necessary steps to be taken to reduce embodied carbon in their projects.
- The AIA Architect’s Guide to Building Performance provides a comprehensive approach to integrating building performance simulations into the design process.
- Energy Star Target Finder is a benchmarking tool for new buildings, updated to use a more recent data set (CBECS 2012+) as a baseline.
- Climate Consultant is a great resource for climate analysis from UCLA. This simple program will generate a variety of climate graphics based on EnergyPlus Weather (.EPW) files and help the user visualize opportunities for the most effective passive strategies.
- PV Watts calculates potential for on-site solar generation and required areas. This is the only solar energy tool you’ll ever need.
- ASHRAE Advanced Energy Design Guides list values of wall insulation, window solar heat gain coefficients (SHGC), and other metrics for project teams looking for effective benchmarks above code.
- 2030 Palette is a free online platform that succinctly puts the principles and actions behind Zero Net Carbon and resilient built environments at the fingertips of designers, planners, and builders worldwide. The swatches (or sustainable design strategies) address energy consumption and greenhouse gas emission at all scales—from regional planning to building details.
- Off-site Procurement of Renewable Energy is a technical support document of Architecture 2030's ZERO Code describing several potential options for off-site procurement of renewable energy.
Design excellence case studies
Explore four COTE® Top Ten award recipients demonstrating successful energy design.
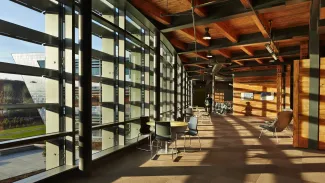
Federal Center South Building 1202
Seattle, Washington | ZGF Architects, Seattle
This office building replaced the conventional building systems with efficient hydronic heating and cooling. This allows the systems to perform at an optimal capacity
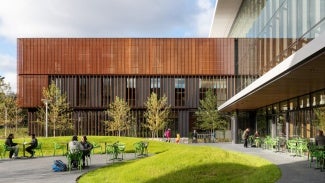
Amherst College New Science Center
Amherst, Massachusetts | Payette
This high-intensity laboratory achieves a low-energy footprint for its typology using a high-performance envelope, low-energy chilled beams, optimized fume hood controls, and various heat recovery strategies to reduce the peak loads and annual energy consumption of the building.
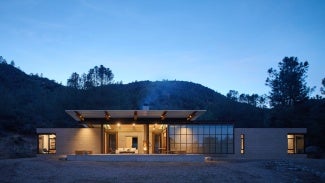
Sawmill
Tehachapi, California | Olson Kundig
This single-family home is self-sufficient; utilizing thermal mass and natural ventilation to reduce the demand on the radiant slab powered by ground source heat pumps that are integrated into a solar array with battery storage.
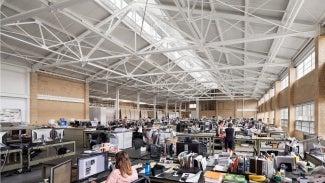
Ortlieb's Bottling House
Philadelphia | KieranTimberlake
The adaptive reuse of a historic urban building that houses an architecture firm reduces the building’s reliance on mechanical systems by 70% with passive strategies that include daylight, thermal mass, and operable windows. Focus topics: passive design strategies, energy benchmarking and goal setting, energy modeling.
This publication is designed to provide accurate and authoritative information in regard to the subject matter covered. It is published and distributed with the understanding that the publisher is not engaged in rendering professional services. If professional advice or other expert assistance is required, the services of a competent professional person should be sought.
AIA does not sponsor or endorse any enterprise, whether public or private, operated for profit. Further, no AIA officer, director, committee member, or employee, or any of its component organizations in his or her official capacity, is permitted to approve, sponsor, endorse, or do anything that may be deemed or construed to be an approval, sponsorship, or endorsement of any material of construction or any method or manner of handling, using, distributing, or dealing in any material or product.
If you have any questions or feedback regarding the Framework for Design Excellence, please let us know.
Learn how the framework inspires and provides a toolkit for sustainable, resilient, and inclusive design.
Explore the next chapter of the Framework for Design Excellence—Design for Well-being.